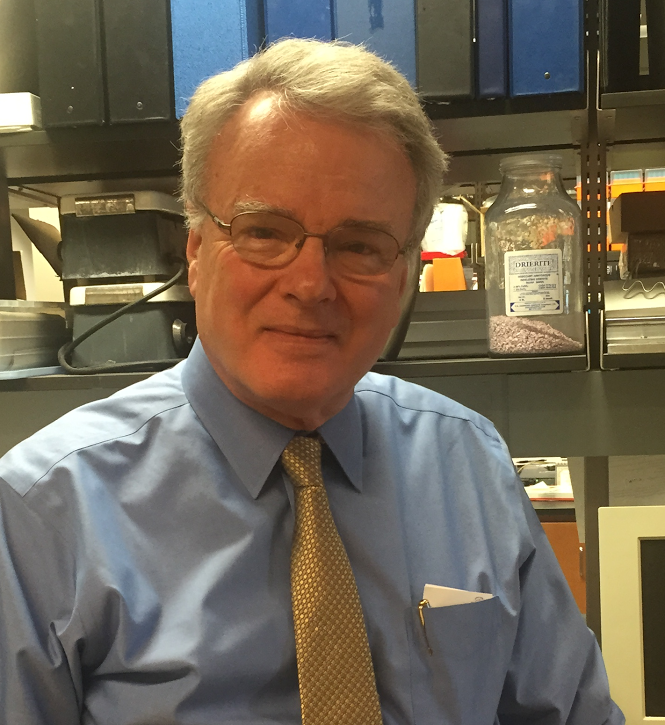
Research
Andersen has a longstanding interest in the biophysical properties of ion channels, where he has done pioneering studies on ion permeation and channel regulation. He developed electrophysiological methods to show: first, that ion entry into membrane-spanning is limited by the aqueous diffusion of the permeating ion up to the channel entrance, which becomes important for understanding the kinetics of ion permeation; and second, that it is possible to determine the structural equivalence of amino acid sequence-substituted ion channels based on their electrophysiological fingerprints, which allows for quantifying the energetic consequences of the substitution(s).
Figure 1
Bilayer-mediated regulation recorded using gramicidin channels, which form by transmembrane dimerization. Bioactive amphiphiles alter/increase bilayer elasticity, thereby promoting channel formation and ion (quencher) flux into flurophore-loaded vesicles, which can be measured using stopped-flow spectrofluorometry. The magnitude of the bilayer-mediated regulation increases with increasing quench rate from low (green) to high (red).
Andersen’s current interest is the regulation of membrane protein function by changes in membrane composition, with focus on the bilayer-mediated regulation of protein by bioactive molecules, drugs for short, which results when drugs partition into lipid bilayers and thereby alter bilayer physical properties. Key tools in these studies are the gramicidin channels, exemplars of membranes proteins that are regulated by changes in membrane composition, as well as probes for quantifying drug-induced changes in membrane properties. His laboratory developed theoretical and experimental tools to quantify how drug partitioning into lipid bilayers alters the bilayer contribution to the free energy cost of membrane protein conformational changes. Using these tools, Andersen and colleagues established the generality of bilayer-mediated regulation of membrane protein and cell function by amphipathic drugs. Importantly, any membrane protein that undergoes conformational changes involving its bilayer-spanning domain will be subject to bilayer-mediated regulation, which in turn may cause dysregulation of cell function—and, if the changes in function are large enough, cell death.
Figure 2
Probability a drug is cytotoxic as function of NormRate (the drug-induced increase in quench rate). The stippled horizantal line denotes the average probability a drug is cytotxic (=0.71).
Indeed, Andersen and colleagues were able to show that a molecule’s (a drug or drug-lead’s) bilayer-modifying potency predicts the probability it will be cytotoxic. This is important in cell physiological studies, where so-called membrane effects are a perennial concern, and in drug development, where knowing a drug-lead’s bilayer-modifying potency may be helpful in guiding medicinal chemistry efforts during the development.
Current Projects:
- Antimicrobial peptides
- Complex membrane proteins
Bio
MD from University of Copenhagen, Denmark in 1971. After postdoctoral training in Biophysics at the University of Copenhagen and The Rockefeller University, Andersen joined the faculty at Weill Cornell in 1973. He became Professor of Physiology and Biophysics in 1982.
Director of the Weill Cornell/Rockefeller/Sloan Kettering Tri-Institutional MD-PhD Program (1996-2021); in 2020 the students in the Tri-Institutional MD-PhD Program established the Olaf S. Andersen Physician-Scientist Award to be given to an MD-PhD student who embodies the values Andersen promoted as Program Director.
Distinctions:
- Member of The Royal Danish Academy of Sciences (1996)
- Recipient of the K. S. Cole Medal (1999)
- Distinguished Service Award (2011) from the Biophysical Society